ISE Fact sheet 2019
Rare Earths – important resources for high technology
High-tech and environmental applications of rare earths have increased dramatically in diversity and importance over the past four decades. Many of these applications are highly specific, since the substitutes for rare earths are inferior or unknown, the rare earths have acquired a technological significance that is much higher than expected due to their relative unknown. Although they are actually more common than many known industrial metals, rare earths tend to focus on non-usable ore deposits. As a result, most of the world’s supply comes from only a few sources. The United States and Europe used to be largely self-sufficient in rare earths, but have become dependent on imports from China over the past decade.
The rare earth elements (rare earths) form the largest chemically coherent group in the periodic table. Although generally unknown, rare earths are essential for many hundreds of applications. The versatility and specificity of rare earths has given them a degree of technological, environmental and economic importance that is considerably higher than one might expect from their relative unfamiliarity. In 1999 and 2000, more than 90 of the rare earths required by US industry came from deposits in China.

Figure 1. Global production of rare earth elements (1 kt = 106 kg) from 1950 to 2000 in four categories: USA, almost exclusively from Mountain Pass, California; China, from several deposits; all other countries together, mostly made of moonitbear-bearing placers; and global sum. Four production periods are recognizable: the Monazite Placer era, which began at the end of the 19th century and ended abruptly in 1964; the Mountain Pass era, which began in 1965 and ended around 1984; a transitional period from about 1984 to 1991; and the Chinese era, which began around 1991. Image source: USGS
Although the 15 naturally occurring rare earths (Table 1; Fig. 2) are generally similar in their geochemical properties, their individual occurrences on Earth are by no means the same. In the continental crust and its rare earth ore deposits, the concentrations of the most common and least common rare earth typically differ by two to five orders of magnitude (Fig. 3). As the technological applications of rare earths have multiplied in recent decades, demand for some of the less common (and formerly quite unchallenged rare earths) has increased dramatically.
The diverse nuclear, metallurgical, chemical, catalytic, electrical, magnetic and optical properties of rare earths have led to an ever-increasing variety of applications. These applications range from banal (lighters, glass polishing) to high-tech (fluorescents, lasers, magnets, batteries, magnetic cooling) to futuristic (high-temperature superconductivity, safe storage and transport of hydrogen for cost-effectiveness) hydrocarbons).
Some rare earth element applications
Many applications of rare earths are characterized by a high specificity and a high unit value. For example, color cathode ray tubes and liquid crystal displays used in computer monitors and televisions use Europium as a red fluorescent; A replacement is not known. Due to the relatively low intake and high demand, the EU is quite valuable – 250 to 1,700 USD/kg (for Eu2O3) in the last decade.
Fiber optic telecommunications cables offer much wider bandwidth than the copper wires and cables they have largely replaced. Fiber optic cables can transmit signals over long distances because they periodically contain spaced lengths of erbium-doped fibers that act as laser amplifiers. It is used in these laser repeaters despite its high cost (700 USD / kg) because it alone has the necessary optical properties.
The specificity is not limited to the more exotic rare earths such as Eu or He. Cer, the most common and cost-effective rare earth, has dozens of applications, some of which are highly specific. For example, Ce-Oxid is uniquely suitable as a polishing agent for glass. The polishing effect of CeO2 depends on both its physical and chemical properties, including the two accessible oxidation stages of Cer, Ce, 3+ and Ce4+, in aqueous solution. Virtually all polished glass products, from ordinary mirrors and glasses to precision glasses, are equipped with CeO2.
Light LREE) |
z | Heavy HREE |
z | |
---|---|---|---|---|
Scandium | 21 | Yttrium | 39 | |
Lanthanum | 57 | Gadolinium | 64 | |
Cerium | 58 | Terbium | 65 | |
Praseodymium | 59 | Dysprosium | 66 | |
Neodymium | 60 | Holmium | 67 | |
Promethium | 61 | Erbium | 68 | |
Samarium | 62 | Thulium | 69 | |
Europium | 63 | Ytterbium | 70 | |
Lutetium | 71 |
Permanent magnet technology has been revolutionized by alloys containing Nd, Sm, Gd, Dy or Pr. Small, lightweight and high-strength rare earth magnets have enabled the miniaturization of numerous electrical and electronic components used in household appliances, audio and video equipment, computers, automobiles, communications systems, and military equipment can be used. Many of the latest technological innovations (such as miniaturized multi-gigabyte portable drives and DVD drives) would not be possible without rare earth magnets.
The environmental applications of rare earths have increased significantly in the last three decades. This trend will undoubtedly continue in the face of growing concern about global warming and energy efficiency. Some rare earths are essential components of both crack catalysts for petroleum fluids and catalysts for controlling automotive pollution. The use of rare earth magnets reduces the weight of motor vehicles. The widespread introduction of new energy-efficient fluorescent lamps (using Y, La, Ce, Eu, Gd and Tb) for institutional lighting could potentially lead to a reduction in carbon dioxide emissions in the US, which is one third of the current emissions in the UNITED States. cars on the road. The large-scale application of magnetic cooling technology (described below) could also significantly reduce energy consumption and CO2 emissions.
Situation in the periodic table
1 h |
2 hey |
||||||||||||||||||||||||||||||
3 Li |
4 mal |
5 b |
6 c |
7 n |
8 o |
9 Q |
10 Ne |
||||||||||||||||||||||||
11 well |
12 Mg |
13 Al |
14 Si |
15 p |
16 see |
17 Cl |
18 Ar |
||||||||||||||||||||||||
19 K |
20 About |
21 Sc |
22 Ti |
23 V |
24 Cr |
25 Mn |
26 Fe |
27 Co |
28 Ni |
29 Cu |
30 Zn |
31 Ga |
32 Ge |
33 As |
34 Se |
35 Bro |
36 Kr |
||||||||||||||
37 Rb |
38 Sr |
39 y |
40 Zr |
41 Nb |
42 Mon |
43 Tc |
44 Ru |
45 Rh |
46 Pd |
47 Ag |
48 Cd |
49 in |
50 Sn |
51 Sb |
52 Te |
53 Ⅰ |
54 Xe |
||||||||||||||
55 Cs |
56 Ba |
57 La |
58 Ce |
59 Pr |
60 Nd |
61 Pm |
62 Sm |
63 Eu |
64 Dg |
65 Tb |
66 Dy |
67 Ho |
68 he |
69 Tm |
70 Yb |
71 Lu |
72 Hf |
73 Ta |
74 w |
75 Re |
76 Os |
77 Ir |
78 Pt |
79 ouch |
80 Hg |
81 Tl |
82 Pb |
83 Bi |
84 Po |
85 At |
86 Rn |
87 Fri |
88 Ra |
89 Ac |
90 Th |
91 Pa |
92 u |
93 Np |
94 Pu |
95 On |
96 Cm |
97 Bk |
98 Cf |
99 it |
100 Fm |
101 Md |
102 № |
103 Lr |
104 Rf |
105 Db |
106 Sg |
107 Bra |
108 Hs |
109 Mt |
110 Ds |
111 Rg |
112 Cn |
113 Nh |
114 Fl |
115 Mc |
116 Lv |
117 Ts |
118 Og |
Figure 2. Chemical periodic table with the 16 rare earth elements (rare earths): the lanthanandes La to Lu and Y, whose geochemical behaviour is practically identical to that of the heavier lanthanoid. Promethium has no long-lived isotopes and is released on Earth only in vanishingly small quantities. An stellt the the first 14 actiniden elements; Lr is the last actinide.
In many applications, rare earths are beneficial due to their relatively low toxicity. For example, the most common types of rechargeable batteries contain either cadmium (Cd) or lead. Rechargeable lanthanum nickel-hydride batteries (La-Ni-H) are gradually replacing Ni-Cd batteries in computer and communications applications and could eventually replace lead-acid batteries in motor vehicles. While La-Ni-H batteries are more expensive, they offer higher energy density, better charging and unloading properties, and fewer environmental problems in disposal or recycling. As another example, red and red-orange pigments produced with La or Ce replace conventional commercial pigments containing Cd or other toxic heavy metals.
The next high-tech application of rare earths to reach maturity could be magnetic cooling. The six rare earth ions Gd3 + to Tm3+ have unusually large magnetic moments due to their multiple unpaired electrons. A newly developed alloy, Gd5 (Si2Ge2), with a „gigantic magnetocaloric effect“ near room temperature will reportedly allow magnetic cooling to become competitive with conventional gas compression cooling. This new technology could be used in refrigerators, freezers and air conditioning systems for households, commercial vehicles and motor vehicles. Magnetic cooling is much more efficient than gas compression cooling and does not require refrigerant that is flammable or toxic, deplete the Earth’s ozone layer, or contribute to global warming.
Rare Earth Occurrences
„Rare“ Earth elements are a historical misnomer. The persistence of the term reflects unfamiliarity rather than true rarity. The most common rare earths are similar in their crust concentration to common industrial metals such as chromium, nickel, copper, zinc, molybdenum, tin, tungsten or lead (Fig. 4). Even the two least common rare earths (Tm, Lu) are almost 200 times more common than gold. Unlike ordinary basic and precious metals, however, rare earths hardly tend to concentrate on usable ore deposits. As a result, most of the world’s rare earth offering comes from only a few sources.
Differences in the frequency of individual rare earths in the upper continental crust of the earth (Fig. 3, 4) represent the superposition of two effects, one nuclear and one geochemical. First, rare earths with even atomic numbers (58Ce, 60Nd,…) have a greater cosmic and terrestrial frequency than neighboring rare earths with odd atomic numbers (57La, 59P,…). Second, the lighter rare earths are more incompatible (because they have larger ion radii) and therefore more concentrated in the continental crust than the heavier rare earths. In most rare earthdeposits, the first four rare earths – La, Ce, Pr and Nd – make up 80 to 99 of the total. Therefore, deposits containing relatively high degrees of scarcer and more valuable heavy rare earths (Heavy Rare Earths: Gd to Lu, Y) and Eu are particularly desirable.
Figure 3. Prices and frequency of rare earth elements. Prices are for 1999 or 2000 in US dollars per Kilogram rare earth metal expressed in two forms: (1) as oxides in packages of 2 to 25 kg with a purity from 95 to 99.99; (2) as 0.1 to 0.45 kg Metal block with a purity of 99.9. two representative rare earth ores - high grade carbonatite ore from Mountain Pass, California, and Laterite ion adsorption ore from southern China - are the earth's upper continental crust. z Atomic number. Image source USGS
diagram showing the frequency of chemical elements in the upper continental crust of the earth as a function of the Atomic number shows Figure 4. Frequency (atom fraction) chemical elements in the upper continental crust earth as a function of the atomic number. Many of the Items are placed in (partially overlapping) categories divided: (1) Rock-forming elements (main elements in the green area and secondary elements in the light green area); (2) Rare earth elements (lanthanides, La-Lu and Y; marked in blue); (3) Main industrial metals (worldwide
production of 3x107 kg / year; printed in bold); (4) Precious metals (italics); and (5) the nine rarest "Metals" - the six elements of the platinum group plus Au, Re and Te (a metalloid). Image source: USGS
Rare Earth Element Resources
From the discovery of rare earths (in the period 1794-1907) to the mid-1950s, some of the rare earths were produced in modest quantities from monazite-containing placers and veins, pegmatite and carbonatites, as well as by-products of uranium and Niobial extraction. During this time, the medium and heavy rare earths were generally available in pure form only in quantities of less than one kilogram and were mainly chemical curiosities.
In 1949, a carbonatite attack with an exceptional content of light rare earths (8 to 12 rare earth oxides [REO]) was discovered at Mountain Pass in California’s upper Mojave Desert (Fig. 5). The rare earths at the mountain pass are mainly from Bastnäsit, (Ce, La, Nd,…) CO3F and related minerals. Until 1966, this single, first-class deposit (owned by Molycorp, Inc.) was the main source of rare earths in the United States. The early development was largely supported by the sudden demand for the EU generated by the commercialisation of colour television. With an average content of 9.3 tonnes (Mt) REO (with a limit of 5), the mountain pass is the only large ore deposit to be mined solely on the basis of its rare earth content. The mountain pass ore is strongly dominated by the light rare earth (Fig. 3, 6). Nevertheless, the large quantities of processed ore and the development of solvent extraction techniques for the large-scale separation of individual rare earths from each other enabled the extraction of several medium rare earths. Increased availability, in turn, led to applications for these formerly exotic elements.
From 1965 to the mid-1980s, Mountain Pass was the dominant source of rare earths, and the United States and Europe were largely self-sufficient in rare earths. Since 1985, the production of rare earths in China has increased dramatically (Fig. 1). Chinese rare earth production comes mainly from two sources. The most important is the iron niobiob rare earth deposit Bayan Obo in Inner Mongolia. This deposit has geological affinities with both carbonatite rare earth deposits and hydrothermal iron oxide (Cu-Au rare earth) deposits such as Olympic Dam, Australia, and Kiruna, Sweden. The grades at Bayan Obo are 3 to 6 REO; Reserves are at least 40 Mt, possibly considerably more. The second main source of Chinese rare earths is ion adsorption in lateritic weathering crusts developed on granite and syenite rocks in tropical south China. These oxidous ores are characterized by a relatively high proportion of heavy rare earths (Fig. 6) and in particular by a simple extraction of rare earths.
The number of editable rare earth deposits, which are already severely restricted by the geochemical properties of rare earths, has also been influenced by environmental and regulatory factors in recent years. Monazite, the most common rare earth mineral, generally contains elevated thorium levels. Th itself is only weakly radioactive, but is accompanied by highly radioactive daughter intermediates, especially radium, which can accumulate during processing. Concern about the risk of radioactivity has largely eliminated monazite as a significant source of rare earths and has focused on the few deposits in which rare earths are present in other low-th-containing minerals, in particular bastnäsite Occurs.
Problems in supplying rare earth elements
In recent years, the only domestic source of rare earths, the mine in Mountain Pass, California, has been sporadically busy. Due to environmental and regulatory problems with the main wastewater pipeline, the Rare Earth Separation Plant (solvent extraction) was shut down. Mountain Pass currently only produces bastnäsite concentrates and sells separate rare earths only from stock produced before the standstill. Even after the regulatory situation has been clarified, the long-term viability of Mountain Pass as a provider of separate rare earths for high-tech applications is threatened by market factors.
Photo with the rare earth element mine Mountain Pass
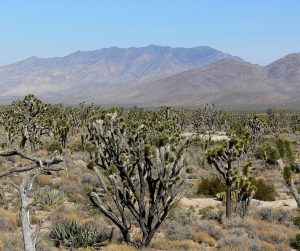
Molycorp Mine, Clark Mountain
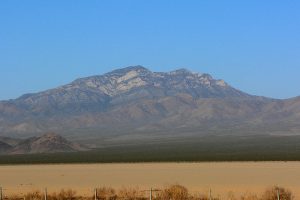
Clark Mountain
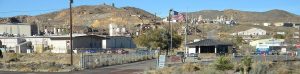
Molycorp Mine
In 1999 and 2000, almost all (more than 90) of the separate rare earths used in the United States and Europe were imported either directly from China or from countries that imported their plant feed from China. The surprisingly rapid transition from self-sufficiency before about 1990 to an almost complete dependence on imports from a single country is now associated with a number of causal factors. This includes much lower labor and regulatory costs in China than in the United States and Europe. Continuation of the expansion of the electronics and other manufacturing industries in Asia; the favourable number, size and favorable heavy rare earth content of Chinese deposits; and the ongoing environmental and regulatory problems at the Mountain Pass. China now dominates the world’s rare earth markets (Fig. 1) and raises some important rare earth supply questions for the United States and Europe and the EU:
(1) The United States and Europe are in danger of losing their long-standing leadership in many areas of rare earth technology. By transferring expertise in rare earth processing technology and rare earth applications from the US and Europe to Asia, China has been able to build a significant rare earth industry that all other countries are able to produce ore and refined products. China’s Ministry of Science and Technology recently announced a new national basic research program. Among the first 15 projects to be financed was „Basic Research for Rare Earth Materials“ (Science, 18 December 1998, p. 2171).
2. The US dependence on imports from China shall occur at a time when rare earths in defence applications, including jet fighter engines and other aircraft components, missile guidance systems, electronic countermeasures, detection of underwater mines, missile defense, distance measurement, space-based satellite power and communications systems have become increasingly important.
(3) The availability of Chinese rare earths to US markets depends on the continued stability of China’s domestic policy and economy, as well as on its relations with other countries.
(4) Although the current low rare earth prices, caused by the abundant supply from China, are weighing on producers, in particular the Molycorp Mountain Pass, low prices are also encouraging the development of new applications. For example, a recent chemistry text states that „… for many years the main application of Lutetium was the study of the behavior of Lutetium…“ Several promising applications for Lu are known, but most are associated with high costs. If the price of Lu were to fall from many thousands of dollars per kilogram (Fig. 3), additional high-tech applications would undoubtedly follow, even for these least common rare earths. How large the role of the United States and Europe in the future expansion of rare earth technology and markets remains an important but open question.
Figure 6. Shares of individual rare earths in two representative ores: Bastnäsit, dominated by La, Ce and Nd, where Eu to Lu plus Y are only 0.4; and lateritic ion adsorption ore, Y-dominated. Dark blue and light blue sectors stand for Lanthanoide with straight or odd ore or atomic number (see Figs. 2, 3). Yttrium is marked in green. Image source: USGS
The rare earth elements are essential for a diverse and growing range of high-tech applications that make up an important part of the industrial economy of the United States and Europe. Long-term shortage or unavailability of rare earths would force significant changes in many technological aspects of Western society. Known and potential domestic rare earth sources can therefore become an increasingly important issue for scientists and policy makers in the public and private sectors.
Sources: US Geological Survey | Geological Department | Mineral Resources Team | Ministry of the Interior | Roskill | Institute of Rare Earths and Metals